The R+D CSIC magazine echoes the scientific study, carried out by CEAB researchers and published in Nature Communications, which reveals the great weight of the environment in the genetic adaptation of bacteria. R+D delves into this research and its possible applications.
(below, reproduction of the article published this January 25 in R+D CSIC, signed by Comunicación CSIC Catalunya)
Knowing how bacteria adapt to their environment opens promising reserach avenues in biotechnology, biomedicine, or the environment. A study from Advanced Studies Center of Blanes (CEAB, one of CSIC’s research institutes) shows that a nutrient-rich environment favors bacteria that have lost genes involved in processes such as amino acid self-production. Dispensing with genes represents an energy-saving for them and gives them an advantage in those contexts.
A study from CEAB reveals that the majority of bacteria (80%) can synthesize the necessary amino acids for their survival on their own. The remaining 20% cannot do so and depend on interaction with other living beings or the environment to obtain them. These are called “auxotrophic” organisms, which need to acquire essential compounds for survival through their diet (similar to humans).
The research shows that auxotrophic bacteria thrive in environments rich in amino acids, such as the human intestine or dairy products (cheese, yogurt, etc.). These environments, abundant in nutrients, promote the evolution of bacteria towards the loss of genes responsible for producing amino acids (which form the basis of their ‘diet’).
Josep Ramoneda, a researcher at the Advanced Studies Center of Blanes (CEAB-CSIC) and the lead author of the article, explains that the reason is simple: “Why to make an effort when you have everything within reach? Having permanent access to a ‘free buffet’ makes self-production of amino acids no longer an advantage.” Ramoneda adds that these environments have favoured a genome optimization strategy where bacteria eliminate unnecessary genes. This helps bacteria to minimize energy costs and thrive. An example are mycoplasmas – bacteria that cause infections in the respiratory and urinary/genital systems – implicated in numerous diseases, which obtain amino acids from the cells of the humans they parasitize.
As the study reveals, bacteria that obtain their resources from the environment have smaller genomes than those that are self-sufficient. They lack genes for other metabolic processes, such as those associated with sugars or lipids. Scientists suggest that this may be a broader genome rationalization strategy, representing significant energy savings for bacteria and being predominant in nutrient-rich ecosystems. On the contrary, in most environments (especially in nutrient-poor or extreme environments), self-sufficiency remains the best option.
Emilio O. Casamayor, a researcher at CEAB-CSIC and co-author of the study, notes, “We have shown that in aquatic environments, such as high mountain lakes or the ocean, where finding food is more challenging or intermittent, the strategy of genome rationalization is not successful. These environments favour bacteria that are self-sufficient.”
Casamayor adds, “Our work has been possible through the combination of massive sequencing and computational techniques, allowing us to undertake studies in predictive ecology at large scales in the microbial world, approaches that were inaccessible for us until very recently.”
The scientists analysed over 26,000 bacterial genomes and 3,800 metagenomes from natural environments such as soils, oceans, lakes, water treatment plants, human and plant microbiota, and foods like sourdough or cheese. The aim was to correlate genes, survival strategies, and the environment.
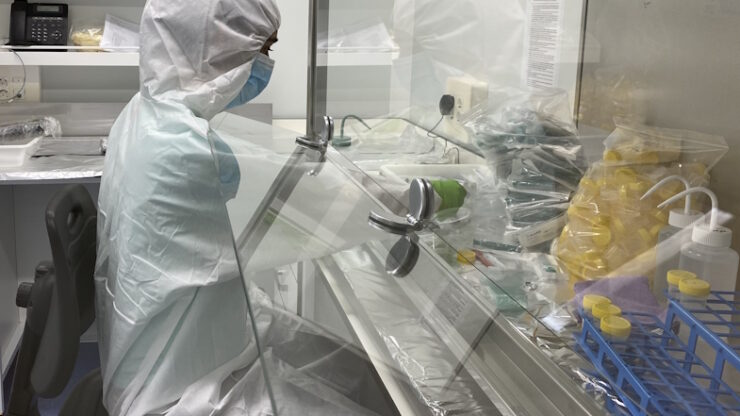
Biotechnological, agricultural, environmental applications…
The resulting models “improve the understanding of the conditions required by many microorganisms that have not yet been successfully cultured in the laboratory” explain the scientists. This has significant biological, genetic, and chemical potential. Among them, there are examples of new drugs, such as rapamycin, derived from a Streptomyces bacterium found on Easter Island in the 1970s.
There are also applications in the field of microbiomes and microbial inoculants for agriculture. “Many microbial cocktail inoculants in agriculture fall short of expectations because they adapt poorly and have poor survival in the field,” say the scientists. “Our predictions can aid in the more efficient development of inoculants. This is also of interest for the effective design of human probiotics.”
On the other hand, “there are numerous microbial species for which we lack information; our models, describing the functional traits of microbial species, could open the door to discovering new organisms or metabolisms of biotechnological interest.”
From an environmental perspective, the research can be useful for the prediction and control of invasions, a well-established practice in animals and plants but significantly lagging behind in microbes, according to scientists. If the environmental preferences of microorganisms are known, models for the distribution of specific species can be established, or ecological invasions, such as red tides or plant pathogens, can be predicted. “We are very aware of macro invasive species, but nothing on a micro scale,” points out Josep Ramoneda.
In addition to CEAB-CSIC, the scientific article, published in Nature Communications, involves researchers from the University of Colorado (USA), Aalborg University (Denmark), and Lawrence Berkeley Lab (USA).